Science & Technology Overview
Nuclear Reactor Sustainment and Expanded Deployment
As the Department of Energy’s (DOE) nuclear energy laboratory, INL is creating and defining the next phase of nuclear energy. INL advances global competitiveness in nuclear energy by sustaining and extending the safe and efficient operation of existing reactors and by pioneering advanced reactor technologies for future deployment. INL’s research, development and demonstration of innovative technologies improve the existing and future nuclear energy systems performance.
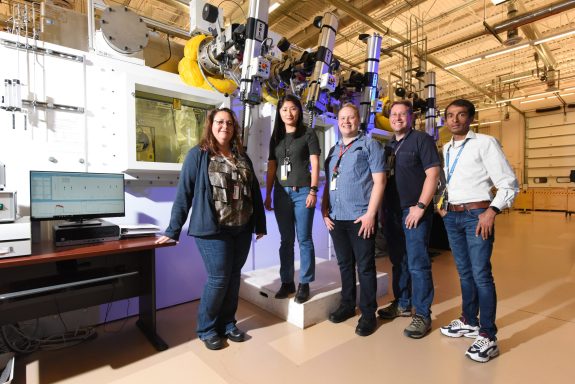
Lab performs post-irradiation examination experiments on
nuclear materials within shielded enclosures.
The nation’s existing fleet of nuclear power plants is essential for fighting climate change. INL provides the technical foundations to strengthen domestic commercial nuclear energy. Working closely with industry and other national laboratories, INL leads research to ensure the economic competitiveness, safety and fuel performance of operating nuclear plants in current and future energy markets. Examples of INL’s industry collaboration include the Accident Tolerant Fuels Program and a first-of-a-kind upgrade of a U.S. nuclear power station to a fully digital system that will provide automation, decrease system interruptions, improve performance and reduce costs.
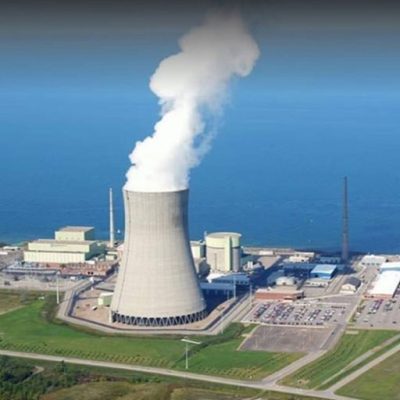
Point Nuclear Station. Photo credit Constellation.
INL produces cutting-edge innovations that fit competitive market niches, foster U.S. economic opportunity and influence the global energy market. Many of INL’s research programs will revolutionize nuclear energy markets. For example, INL is innovating ways to transform nuclear systems into fission batteries. A fission battery has minimal site requirements; is mobile; is safe, secure and autonomous; has a standardized, scalable form factor; and is a cost-effective option for grid and off-grid energy demands. During a recent demonstration at the micro agile non-nuclear experimental testbed (MAGNET) facility, a digital twin of MAGNET successfully achieved autonomous control of MAGNET to predict future heat pipe temperatures. This is a key step toward enabling self-adjusting, cost-efficient and safe microreactors.
INL also plays a crucial role in deploying nuclear technology in space, working with NASA to deliver radioisotope power systems for rovers and the Pluto New Horizons spacecraft. INL is fueling and testing the multi-mission thermoelectric generator (MMRTG) that will power and provide heat for Dragonfly, a drone designed with advanced instrumentation to study Saturn’s largest moon, Titan. That mission is scheduled to launch in 2026. In addition, INL is developing a fission surface power source for NASA missions to the moon by the end of this decade.
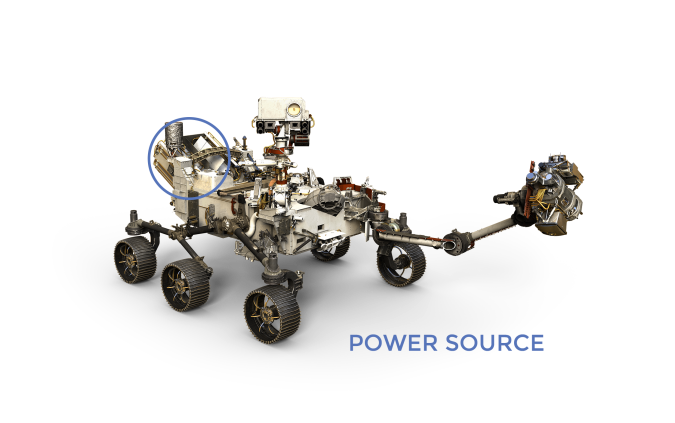
Point Nuclear Station. Photo credit Constellation.
As the lead DOE laboratory for the Department of Defense mobile microreactor program called Pele, INL is managing the design, development and demonstration of a microreactor to power military bases and other critical military infrastructure. The Pele microreactor prototype, which is in the design phase, will be located at INL. To accelerate advanced reactor development and deployment, INL will host the Microreactor Applications Research Validation and Evaluation (MARVEL) reactor, the first highly anticipated demonstration of an advanced reactor technology. MARVEL is an experimental reactor that will enable microreactor applications testing, regulatory approval process development, remote monitoring systems evaluation, and autonomous control technology development. In 2022, the primary coolant acceptance test (PCAT) assembly was completed. PCAT is a full-scale, electrically heated prototype of MARVEL that will provide critical understanding of fundamental microreactor features, operation and behavior. MARVEL’s construction will begin in 2023, and reactor operation is anticipated in 2024.
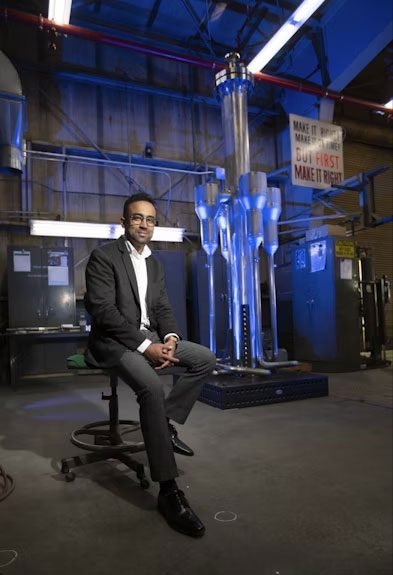
at the Materials and Fuels Complex Fabrication Shop.
Advanced reactor concepts benefit from unique fuel systems, and INL is the primary driver of research programs developing and qualifying several core technologies required to enhance the current reactor fleet and enable advanced reactor deployment. In collaboration with others, INL developed and is qualifying the new TRISO fuel, which is the choice for many advanced reactor concepts.
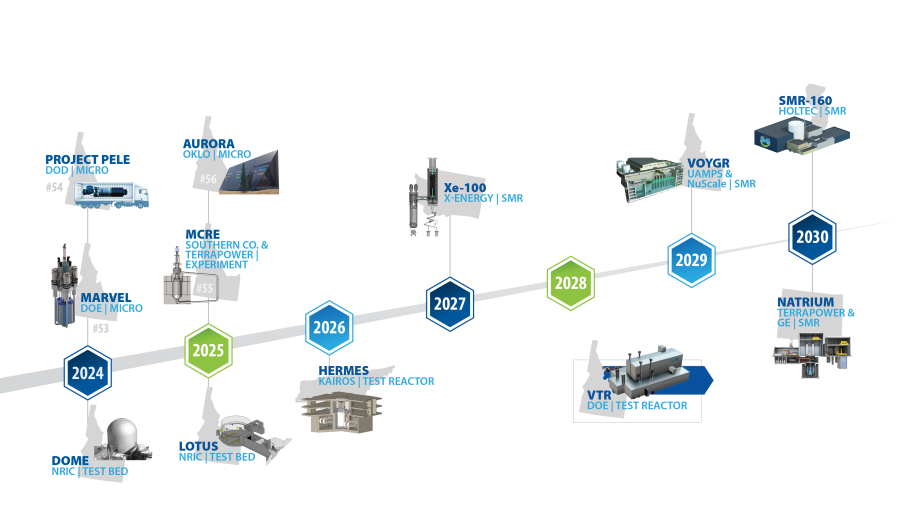
Fundamental research is essential to advance nuclear technology. INL’s portfolio of DOE Basic Energy Sciences projects supports a variety of nuclear-related research. INL’s Energy Frontiers Research Center for Thermal Energy Transport under Irradiation (TETI) is a multi-institutional program developing a comprehensive, atom-to-microstructure scale understanding of phonon- and electron-mediated transport in nuclear fuels. Success relies on utilizing new post-irradiation characterization capabilities developed from significant laboratory and Office of Nuclear Energy investments.
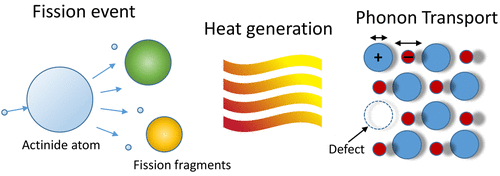
Fission fragments create damage and are deposited as chemical impurities.
Chem. Rev. 2022, 122, 3, 3711-3762.
Ensuring advanced reactor design and deployment and sustaining the current fleet require dedicated infrastructure able to meet shifting energy needs. Advanced reactor demonstrations, fuel development and irradiation testing capabilities are just a few examples highlighting the need for strategic infrastructures to expand the U.S. nuclear energy industry.
INL provides state-of-the-art examination and characterization facilities to accelerate development and qualification of advanced reactor fuels and materials. The Hot Fuel Examination Facility, with the largest inert atmosphere hot cell in the U.S., is equipped to handle both destructive and nondestructive examinations of irradiated fuel ranging from commercial light water reactor fuel rods to TRISO fuel particles. The Irradiated Materials Characterization Laboratory offers cutting-edge resources for advanced post-irradiation examinations to understand material behavior at the micro- and nanostructural level through microstructural, thermal and mechanical characterization of irradiated nuclear fuels and materials. An additional hot cell characterization facility under construction at the Materials and Fuels Complex, the Sample Preparation Laboratory, will accommodate high throughput testing and analysis of reactor structural materials. The lab will begin radiological operations in 2026 and is anticipated to accelerate research required to sustain the current fleet and develop advanced nuclear innovations.
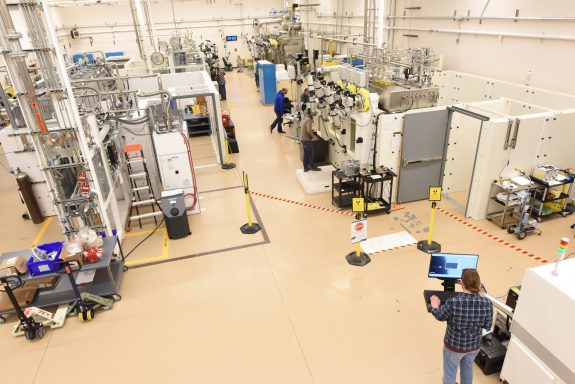
instrumentation for post-irradiation examination experiments.
INL extends nuclear materials and fuel cycle capabilities through the INL-developed Multiphysics Object Oriented Simulation Environment (MOOSE) application. MOOSE and the MOOSE-based applications substantially improve design, analysis and validation of new technologies, approaches and experiments to expedite development and qualification of new nuclear technologies.
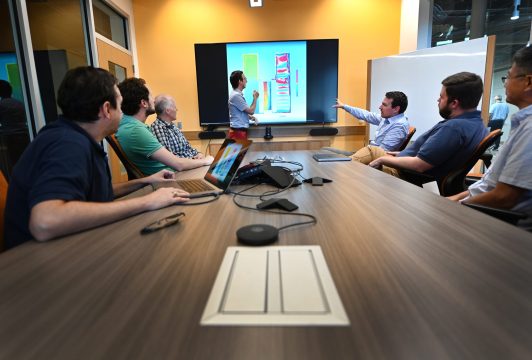
INL’s 890-square-mile site hosts world-class facilities providing strategic irradiation capabilities for the nation. Testing irradiated nuclear reactor materials is crucial to advance nuclear energy. The Advanced Test Reactor (ATR), operated and maintained by the Office of Nuclear Energy at INL, is the sole test reactor in the U.S. capable of providing large-volume, thermal irradiation testing. In 2022, ATR underwent its sixth Core Internals Changeout (CIC), which is vital to its operation through 2040, along with additional retrofits to expand irradiation capabilities. Learn more about the CIC process.
INL’s one-of-a-kind Transient Reactor Test (TREAT) Facility also offers unique and versatile testing capabilities. Unlike ATR, the TREAT facility uses controlled, short-term bursts of intense neutron flux to heat fuel specimens and simulate hypothetical accidents in nuclear power plants. Like car crash testing for nuclear fuel, TREAT uses advanced instrumentation to capture dynamic data related to material and fuel behavior under challenging conditions. The facility uses different capsules and loops with pressurized water, liquid metals or gas environments to simulate several reactor environments.
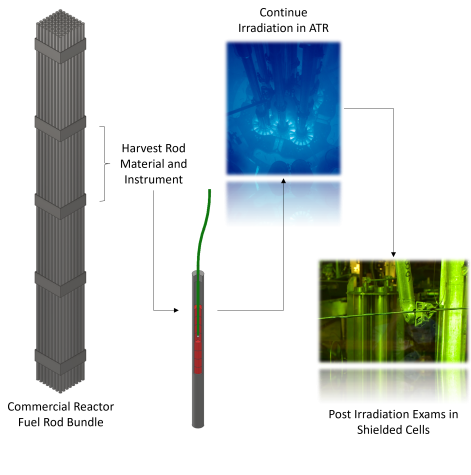
left by closure of the Halden Boiling Water Reactor in Norway to be reclaimed at ATR.
Like ATR, recent developments at TREAT have focused on reclaiming the capabilities lost by the closure of the Halden Boiling Water Reactor in Norway. Supporting industry goals to extend the burn-up of current fuels, advancements include the Transient Water Irradiation System (TWIST). This unparalleled light water reactor fuel safety device will synchronize TREAT’s power control with rapidly draining water, creating high-temperature conditions in test rods to investigate high burn-up fuel performance during hypothetical loss of coolant accidents.
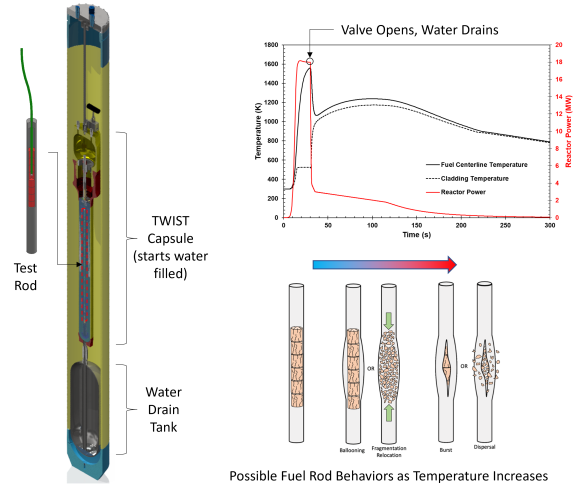
conditions on a test rod in TREAT.
The INL Measurement Science Laboratories are a collection of laboratory spaces, equipment and capabilities that provide broad support to many programs within the U.S. Department of Energy’s Office of Nuclear Energy allowing access to researchers and engineers from organizations inside and outside INL. Instrumentation research, development and deployment activities at the Measurement Science Laboratories leverage the portfolio of DOE’s Nuclear Energy Enabling Technologies Advanced Sensors and Instrumentation program as needed to meet customer needs, which include developing and fabricating nuclear instrumentation, engineering services for instrumented irradiation rigs, and developing innovative sensing technologies.
ADVANCED COMPUTER SCIENCE, VISUALIZATION, AND DATA
The ability to have a widely recognized role in advances in all applications in computational science and engineering. A core capability in these areas would involve expertise in areas such as programming languages, high-performance computing tools, peta- to exa-scale scientific data management and scientific visualization, distributed computing infrastructure, programming models for novel computer architectures, and automatic tuning for improving code performance, with unique and/or world-leading components in one or more of these areas. The capability requires access to (note: these resources do not need to be co-located) a high-end computational facility with the resources to test and develop new tools, libraries, languages, etc. In addition, linkages to application teams in computational science and/or engineering of interest to the Department of Energy and/or other Federal agencies would be beneficial to promptly address needs and requirements of those teams.
APPLIED MATERIALS SCIENCE AND ENGINEERING
The ability to conduct theoretical, experimental, and computational research to understand and characterize materials with focus on the design, synthesis, scale-up, prediction and measurement of structure/property relationships, the role of defects in controlling properties, the performance of materials in hostile environments to include mechanical behavior and long-term environmental stability, and the large-scale production of new materials with specific properties. The strong linkages with molecular science, engineering, and environmental science provides a basis for the development of materials that improve the efficiency, economy, cost-effectiveness, environmental acceptability, and safety in energy generation, conversion, transmission, and end-use technologies and systems. Primary supporting disciplines and field include materials synthesis, characterization, and processing; chemical and electrochemistry, combinatorial chemistry, surface science, catalysis, analytical and molecular science; and computation science.
BIOLOGICAL AND BIOPROCESS ENGINEERING
Applies understanding of complex biological systems and phenomena to design, prototype, test and validate processes components, technologies, and systems relevant to (1) bioenergy production, (2) environmental contaminants processing, and (3) global carbon cycling and biosequestration. Primary supporting disciplines include chemical engineering, agricultural science, fermentation science, materials science and engineering, and systems science.
CHEMICAL AND MOLECULAR SCIENCE (EMERGING)
The ability to conduct experimental, theoretical, and computational research to fundamentally understand chemical change and energy flow in molecular systems that provide a basis for the development of new processes for the generation, storage, and use of energy and for mitigation of the environmental impacts of energy use. Areas of research include atomic, molecular, and optical sciences; gas-phase chemical physics; condensed phase and interfacial molecular science; solar photochemistry; photosynthetic systems; physical biosciences; catalysis science; separation science; actinide chemistry; and geosciences.
CHEMICAL ENGINEERING
The ability to conduct applied chemical research that spans multiple scales from the molecular to macroscopic and from picoseconds to years. Chemical engineering translates scientific discovery into transformational solutions for advanced energy systems and other U.S. needs related to environment, security, and national competitiveness. The strong linkages between molecular, biological, and materials sciences, engineering science, and separations, catalysis and other chemical conversions provide a basis for the development of chemical processes that improve the efficiency, economy, competitiveness, environmental acceptability, and safety in energy generation, conversion, and utilization. A core capability in chemical engineering would underpin R&D in various areas such as nanomanufacturing, process intensification, biomass utilization, radiochemical processing, dielectric materials, advanced conducting materials, high-efficiency clean combustion, and would generate innovative solutions in alternative energy systems, carbon management, energy-intensive industrial processing, nuclear fuel cycle development, and waste and environmental management.
CONDENSED MATTER PHYSICS AND MATERIALS SCIENCE (EMERGING)
The ability to conduct experimental, theoretical, and computational research to fundamentally understand condensed matter physics and materials sciences that provide a basis for the development of materials that improve the efficiency, economy, environmental acceptability, and safety in energy generation, conversion, transmission, and utilization. Areas of research include experimental and theoretical condensed matter physics, x-ray and neutron scattering, electron and scanning probe microscopies, ultrafast materials science, physical and mechanical behavior of materials, radiation effects in materials, materials chemistry, and bimolecular materials.
CYBER AND INFORMATION SCIENCES
The disciplines, technologies, and practices designed to protect, analyze, and disseminate information from electronic sources, including computer systems, computer networks, and sensor networks. A core competency in this area would involve recognized expertise in one or more of the following topics: cyber security, information assurance, information analytics, knowledge representation, and information theory, control systems design and engineering, embedded systems, reverse engineering, and advanced hacking techniques. This core competency would be applied to: the protection of information systems and data from theft or attack; the collection, classification, analysis, and sharing of disparate data; and the creation of knowledge from heterogeneous information sources; securing control systems integrated into critical infrastructure; and increasing security, reliability, and resilience of automated processes and systems.
DECISION SCIENCE AND ANALYSIS
Derives knowledge and insights from measured and modeled data sets to further the understanding of and tradeoffs among resource and technology options, to identify and quantify the risks and impacts of current and emerging technologies on environmental systems, and to assess the impact of market dynamics, human behavior and regulations, policies or institutional practices on the development and uptake of technology. Primary supporting disciplines include engineering, environmental science, applied math, finance, business, social and political science, and market and behavioral economics. This capability provides credible and objective information to support DOE and others to support strategic planning and program direction, policy formulation and implementation, efforts to remove market barriers to deployment and engagement with stakeholders.
EARTH, ENVIRONMENTAL, AND ATMOSPHERIC SCIENCE
The ability to understand and predict the physical, chemical, and biological structure and function of subsurface environments to enable systems-level environmental prediction and decision support related to the sustainable development of subsurface resources, environmentally-responsible use of the subsurface for storage, and effective, mitigation of the impacts of environmental contamination from past nuclear weapons production and provide a scientific basis for the long-term stewardship of nuclear waste disposal via the integration of laboratory-specific research facilities, instrumentation and/or leadership-class computational systems, and multidisciplinary teams of individuals with expertise in environmental subsurface science and related disciplines in microbial ecology and biogeochemistry. This unique combination of tools and expertise is the foundation for research on (1) linking research across scales from the molecular to field scale, (2) integration of advanced computer models into the research and (3) multidisciplinary, iterative experimentation to understand and nutrient cycling and contaminant transport in complex subsurface environments. This ability can contribute to mitigating the impacts of environmental contamination from past nuclear weapons production and provide a scientific basis for the long-term stewardship of nuclear waste disposal, as well as understanding subsurface environments and their role in the functioning of terrestrial ecosystems.
LARGE-SCALE USER FACILITIES/ R&D FACILITIES/ADVANCED INSTRUMENTATION
The ability to conceive, design, construct and operate leading-edge specialty research facilities available to universities, industry, and national laboratories customers to conduct groundbreaking research and development activities and/or ‘at scale’ testing and demonstration of technology. This includes the ability to effectively manage construction of $100 million or greater one-of-a-kind scientific facilities, and to host hundreds to thousands of U.S. and international users in addition to carrying out world-class research at the facility itself. The ability to conceive, design, build, operate and use first-in-class technical instruments intended for a particular research purpose, often requiring the material expertise of multiple scientific disciplines. Instrumentation that can be created by a small number of individuals or that would sit on a laboratory benchtop is not considered part of this core capability.
MECHANICAL DESIGN AND ENGINEERING
Applies the principles of physics, mechanics, and materials science to analyze, design, test, validate, and enable operation of advanced engineered systems, machines, and tools. Includes equipment used to move or extract energy bearing materials (e.g., oil, gas, coal) or from moving fluids (e.g., water, wind, steam), as well as equipment used to convert energy to useful services (e.g., mobility, home heating and cooling, robotics, imaging devices, etc.) or to manufacture products. Primary supporting disciplines include physics, materials science, aerospace engineering, mechanical engineering, chemical engineering, electrical engineering, and computational science.
NUCLEAR AND RADIOCHEMISTRY
The ability to use a broad range of facilities, instrumentation, equipment and, often, interdisciplinary teams that apply the knowledge, data, methods, and techniques of nuclear chemistry, mechanical engineering, chemical engineering to missions of the Departments of Energy and Homeland Security. The elements of this capability are often brought together in unique combinations with those of other disciplines to address high priority needs such as new and improved nuclear systems; radioisotope production and advanced instrumentation for nuclear medicine; development of methods and systems to assure nonproliferation and combat terrorism; and environmental studies, monitoring, and remediation.
NUCLEAR ENGINEERING
The ability to use a broad range of facilities, instrumentation, equipment and, often, interdisciplinary teams that apply the knowledge, data, methods, and techniques of nuclear engineering, mechanical engineering, nuclear reactor physics, measurable science, and risk assessment to missions of the Departments of Energy and Homeland Security. The elements of this capability are often brought together in unique combinations with those of other disciplines to address high priority needs such as new and improved energy sources and systems; advanced instrumentation for nuclear systems; accelerator science and technology; and development of methods and systems to assure nonproliferation and combat terrorism.
POWER SYSTEMS AND ELECTRICAL ENGINEERING
Applies understanding of electromagnetic phenomena to design and engineer circuitry, electrical and electronic devices and equipment, sensors, instruments, and control systems to address the efficiency and reliability of power transmission and distribution systems, and the interface of the grid with variable generation and modern loads. Primary supporting disciplines include electrical engineering, power systems engineering, computational science, and materials synthesis, characterization, and processing.
SYSTEMS ENGINEERING AND INTEGRATION
The ability to solve problems holistically from the concept and design phase to ultimate deliverable and completion phase, by synthesizing multiple disciplines, and to develop and implement optimal solutions. The ability to develop solutions that address issues of national energy and environmental security. Areas of application of this capability include development of programs in energy supply, storage, transportation, and efficiency; and deployment of novel solutions to materials and sensor problems in fields of interest to the Department of Energy and/or the Department of Homeland Security.
COMPUTATIONAL SCIENCE
The ability to connect applied mathematics and computer science with research in scientific disciplines (e.g., biological sciences, chemistry, materials, physics, etc.). A core capability in this area involves expertise in applied mathematics, computer science and in scientific domains with a proven record of effectively and efficiently utilizing high performance computing resources to obtain significant results in areas of science and/or engineering of interest to the Department of Energy and/or other Federal agencies. The individual strengths in applied mathematics, computer science and in scientific domains in concert with the strength of the synergy between them is the critical element of this core capability.
ISOTOPE SCIENCE AND ENGINEERING
The ability to produce, process, enrich or separate isotopes for distribution, and the research that enables this core competency. This includes technologies, byproducts, and surplus materials for production and processing of radioisotopes using reactor or accelerator facilities, material separation, or enrichment of radioactive and stable isotopes. The ability to conduct theoretical, experimental, and computational research to develop isotope production, processing, enrichment, and separations capabilities and technologies, and to translate to routine production of new isotopes with specific assays. This core competency may include the production of isotopes following current Good Manufacturing Processes, and knowledge to operate reactors, particle accelerators, processing equipment or enrichment devices. Areas of research needed to develop this core competency include chemical processing, analytical chemistry, nuclear and radiochemical processing, catalysis science, separation science, material science, biology, actinide chemistry, computational science, reactor physics, accelerator science, advanced manufacturing, targetry, mechanical engineering, chemical engineering, robotics, enrichment science and engineering.
PLASMA AND FUSION ENERGY SCIENCES
The ability to control, maintain, and utilize matter in the state of plasma across a range of conditions to conduct world-leading research. This includes low-temperature plasmas which are utilized in applications such as microelectronics fabrication and nanomaterial synthesis and can have significant spinoff applications, and high temperature/high pressure plasmas, also known as burning plasmas, which are critical for developing the scientific foundation for fusion as a future energy source. This ability can be demonstrated across this entire range of plasma conditions in the operation of state-of-the-art experimental facilities that can support research on the fundamental physics of plasmas; in theory and advanced simulations which are critical to the full understanding of the plasma phenomena being studied and on how plasmas can be best controlled and utilized; and in enabling technologies that allow experiments to reach the necessary conditions where new discoveries can be made.