Science & Technology Overview
Foundational R&D to Support Capabilities
A strong science program is paramount to INL’s science and technology initiatives and strategic plan. INL leverages foundational research and development (R&D) programs to support capabilities through its Laboratory Directed Research and Development (LDRD) program and through its Office of Science program. Both programs are continuously growing and route vital scientific competencies into INL’s strategic initiative areas to help bridge the gap between fundamental science and engineering to deliver groundbreaking technologies.
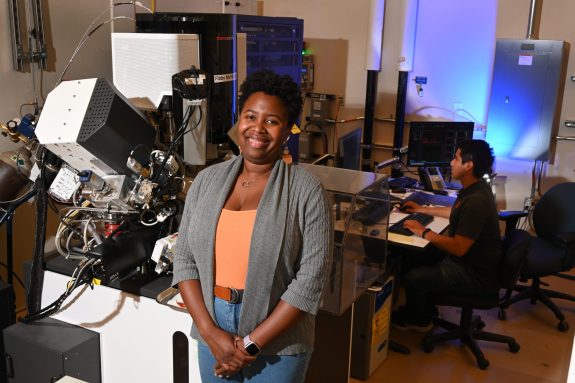
use the focused ion beam for their LDRD project on corrosion studies for
molten salt reactors.
INL’s Office of Science work enables basic research, discovery science and innovation that enable bridging fundamentals with engineering applications. INL’s Office of Science portfolio includes projects in Basic Energy Sciences (BES), core work for tritium safety in Fusion Energy Sciences, and participation in Advanced Scientific Computing Research. Three Office of Science Early Career Researcher Awards in the last eight years demonstrate INL’s commitment to, and success in, developing a vibrant fundamental science program.
INL’s core program in the Materials Science and Engineering Division through BES investigates the formation mechanism of ordered nanostructures under ion irradiation. This has potential applications in energy sciences, ranging from light management in topological photonic structures to increased fission gas retention in advanced nuclear fuels. BES support has enabled significant progress in determining the formation of these related nanostructures. This has spawned new research opportunities including understanding the formation mechanism of ordered nanostructures at cryogenic temperatures and extending this knowledge to more chemically complex ceramic materials.
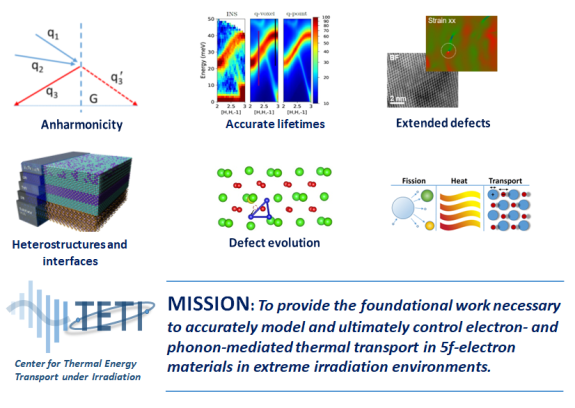
In 2022, INL researchers successfully recompeted for an Energy Frontier Research Center. INL’s current Center for Thermal Energy Transport under Irradiation (TETI), established in 2018, will receive $12.6 million through 2026. Led by INL, TETI brings together researchers from a broad spectrum of research fields and institutions to understand the fundamental physical mechanisms driving heat transfer in advanced nuclear fuels under irradiation, which directly relates to reactor efficiency and safety. Additionally, INL participates in two other Energy Frontier Research Centers:
- Molten Salts in Extreme Environments. Led by Brookhaven National Lab, INL significantly contributes by leveraging its unique facilities for handling radioactive materials to investigate the fundamental chemistry of molten salts in bulk and at the interface.
- Multi-scale Fluid-Solid Interactions in Architected and Natural Materials. Led by University of Utah, INL is helping develop a new fundamental understanding and models of transport and interfacial properties of confined fluids.
INL was recently awarded funding through BES to lead research over the next three years on the fundamental radiation-induced behavior of iodine in molten salts in support of the Chemical and Materials Sciences to Advance Clean Energy Technologies and Low-Carbon Manufacturing initiative. The goal of this initiative is to establish foundational knowledge in research activities to minimize climate impacts of energy technologies and manufacturing.
Supported by Advanced Scientific Computing Research, INL will participate over the next five years in a Scientific Discovery through Advanced Computing project to develop a multiscale capability for predicting both microstructure evolution and mechanical failure of materials simultaneously exposed to an actinide containing salt, irradiation, stress and temperature. INL is implementing a mechanistic surrogate model in the Multiphysics Object-Oriented Simulation Environment (MOOSE) framework to simulate the propagation of a crack under varying loading and microstructure conditions.
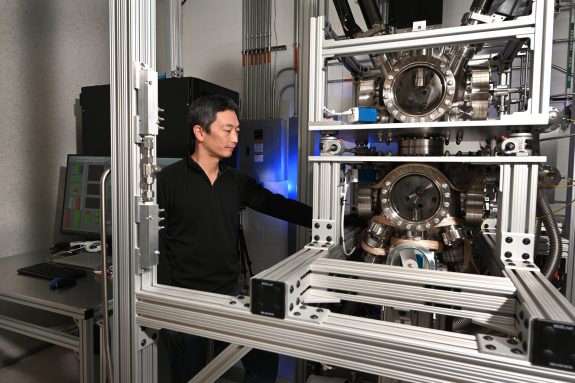
advanced surface characterization techniques to help understand material evolution
in membranes for plasma exhaust processing in magnetic fusion experiments.
INL has been the lead laboratory for magnetic fusion energy safety for the Fusion Energy Sciences program for more than 30 years. INL participated in the Fusion Energy Science Advisory Committee 2020 long-range planning report “Powering the Future: Fusion and Plasmas,” which lays out a decade-long vision and presents a path for the delivery of fusion energy by 2040. INL supports the mission of the Fusion Energy Sciences program in the following computational and experimental areas:
- Continuing development of the MELCOR-fusion code to support integrated system-level tritium transport and accident analyses for licensing fusion reactors.
- Extending computational capabilities for fusion by coupling the Tritium Migration Analysis Program with INL’s MOOSE framework to allow tritium transport and multiphysics modeling in complex tritium breeding blanket systems.
- Providing fundamental tritium transport properties (e.g., diffusivity, solubility, and concentration and binding energy of trap sites) database in fusion reactor materials with tritium-exposed and low-level activated materials at the Safety and Tritium Applied Research (STAR) facility.
- Leading the development of superpermeable metal foil pumps from plasma exhaust and tritium extraction technology from liquid breeding blanket material at the STAR facility for the Blanket and Fuel Cycle program under Fusion Energy Sciences.
The LDRD program offers researchers opportunities to pursue their ideas across INL’s five science and technology initiatives and other strategic areas. LDRD investments stimulate high-impact and high-risk research projects, amplify INL’s core capabilities, and help recruit world-class talent. In 2022, INL’s LDRD program secured $40 million to fund over 200 research projects, hire distinguished postdoctoral candidates and kickstart novel research. Additionally, LDRD investments grow new programs, intellectual property, patents, copyrights, awards, and publications. INL is aggressively growing the LDRD program because it is vital to advancing INL’s mission and R&D strategy.
In 2022, INL funded 16 projects focused on the chemical and molecular science, condensed matter physics and materials science core capabilities. One example is the work of Amey Khanolkar. He was awarded LDRD funding to study lattice anharmonicity in strongly correlated systems via a holistic research plan including computational and experimental methods. He is developing an optical instrument that will measure fundamental physical properties in micrometer-scale single crystals enabling computational predictions on model systems to be validated. This is one of many projects advancing the emerging fields of condensed matter physics, materials science and supporting computational science.
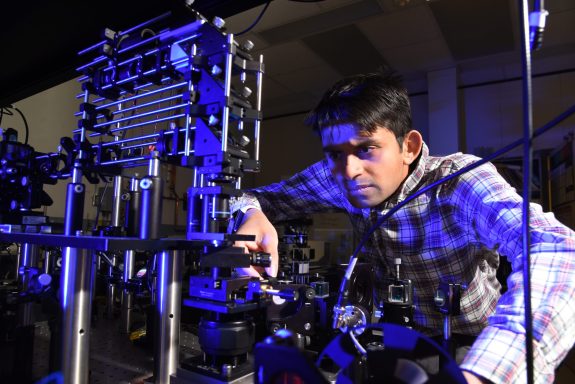
of single crystals for his LDRD project in condensed matter physics.
Thermal Energy Transport in Oxide Nuclear Fuel
As part of TETI, INL researchers and collaborators published a comprehensive review of thermal transport research on two actinide oxides where heat is carried by lattice waves. UO2 is used in current commercial nuclear reactors, and ThO2 is a potential fuel candidate for advanced reactors. The review outlines computational and experimental tools being developed to model and eventually control thermal transport in advanced nuclear fuels and highlights existing research gaps and challenges. This article lays the groundwork for exciting new research directions in nuclear fuels and beyond to other energy-related technologies.
Hurley, D. H., El-Azab, A., Bryan, M. S., Cooper, M. W. D., Dennett, C. A., Gofryk, K., He, L., Khafizov, M., Lander, G. H., Manley, M. E., Mann, J. M., Marianetti, C. A., Rickert, K., Selim, F. A., Tonks, M. R., & Wharry, J. P. (2022). Thermal Energy Transport in Oxide Nuclear Fuel. Chemical Reviews, 122(3), 3711-3762.
Switching of metal-oxygen hybridization for selective CO2 electrohydrogenation under mild temperature and pressure
Funded through INL’s LDRD program, researchers performed electrochemical techniques, material design and characterization, and theoretical calculations to demonstrate how an advanced electrolyzer could be extended to the upgrade of different carbon resources in one step. This research provides new insights in carbon conversion techniques to reduce atmospheric CO2 levels and combat climate change.
Li, M., Hua, B., Wang, L. C., Sugar, J. D., Wu, W., Ding, Y., Li, J., & Ding, D. (2021). Switching of metal–oxygen hybridization for selective CO2 electrohydrogenation under mild temperature and pressure. Nature Catalysis, 4(4), 274-283.
Transient Radiation-Induced Berkelium(III) and Californium(III) Redox Chemistry in Aqueous Solution
Supported through INL’s LDRD program and Basic Energy Sciences funding, researchers demonstrate the existence of complex radiation-induced redox chemistry in aqueous solutions of late actinide ions, a growing area of interest due to their potential applications in many scientific and technological fields. The chemical reaction kinetics measured using transient absorption spectroscopy bridge gaps in our fundamental knowledge of the chemical behavior of late actinides significantly extending the limited information available prior to this study.
Horne, G. P., Rotermund, B. M., Grimes, T. S., Sperling, J. M., Meeker, D. S., Zalupski, P. R., Beck, N., Huffman, Z. K., Martinez, D. G., Beshay, A., Peterman, D. R., Layne, B. H., Johnson, J., Cook, A. R., Albrecht-Schönzart, T. E., & Mezyk, S. P. (2022). Transient Radiation-Induced Berkelium(III) and Californium(III) Redox Chemistry in Aqueous Solution. Inorganic Chemistry, 61(28), 10822-10832.
Surface effects on deuterium permeation through vanadium membranes
Funded through the Fusion Energy Sciences program, INL researchers performed a deuterium permeation study in vanadium, which is a candidate material for various hydrogen separation technologies. Combined with Auger electron spectroscopy analysis, researchers revealed complex interplay between surface oxide layer and subsurface carbide layer that reduces hydrogen permeation in uncoated vanadium. Intermetallic diffusion of palladium and vanadium measured by Auger electron spectroscopy analysis also advances our understanding of surface effects on hydrogen permeation and helps extend the lifetime of palladium-coated vanadium membranes.
Fuerst, T. F., Humrickhouse, P. W., Taylor, C. N., & Shimada, M. (2021). Surface effects on deuterium permeation through vanadium membranes. Journal of Membrane Sciences, 620, 118949.
INL’s 890 square miles make up the Department of Energy’s largest national lab site. The planning, maintenance, operations and modernization groups work in unison with INL’s research, development and demonstration (RD&D) functions to facilitate mission needs. Watch the video to see an aerial view of INL’s campus.
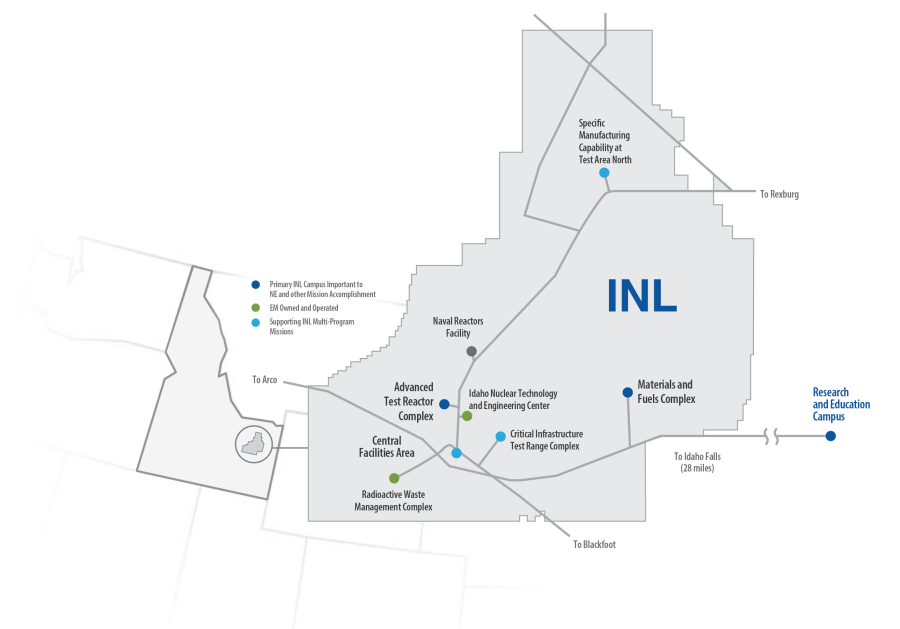
of single crystals for his LDRD project in condensed matter physics.
Advanced Test Reactor team completes the test reactor’s sixth core overhaul: INL’s Advanced Test Reactor (ATR) design accommodates periodic replacement of beryllium reflector blocks and other key components that wear out over time in the reactor’s high neutron and gamma radiation environment. ATR’s sixth overhaul, known as the Core Internals Changeout, or CIC, began in April 2021 and was completed in March 2022. It is the result of over five years of planning and preparations, involving virtually every member of the ATR organization – operations, maintenance, radiological controls, engineering and other support staff members, along with assistance from other INL departments and subcontractor employees. This unique CIC involved replacing more components than in previous overhauls. Extensive preparatory training and inventory efforts for the rarely executed activity were key to administering the outage with minimal delay and less radiation dose than ever before. The overhaul enables ATR to continue its missions supporting the Department of Energy’s (DOE) offices of Nuclear Energy, Naval Reactors, Science and other customers for years to come.
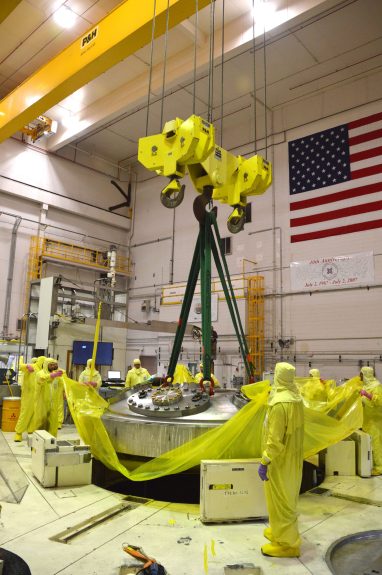
head from the reactor vessel, allowing
access to the core components below.
Upgrades to the Analytical Research Laboratory at the Materials and Fuels Complex: Over the past five years, INL has invested more than $30 million in the physical infrastructure and analytical capabilities of the Materials and Fuels Complex’s Analytical Research Laboratory. Infrastructure improvements, which include a significant upgrade to the heating, ventilation and air conditioning system, installation of an exterior insulation and finishing system, and new energy-efficient windows, allow the laboratory to meet increasing program demands and precise operating parameters of new instrumentation for the coming decade. Laboratory renovations included replacing constant air flow hoods with variable air flow hoods and installing pressure and air flow controls in ducting to maintain greater temperature control, corrosion-resistant drains and piping, and modernizing lab spaces to improve functionality and the working environment. The lab also received state-of-the-art analytical instrumentation. The improvements in precision and resolution provided by these new instruments allow the Analytical Research Laboratory to meet demand by improving reliability, increasing throughput and lowering detection limits. From a new multicollector inductively coupled plasma mass spectrometer to a laser ionization breakdown spectroscopy system to a suite of alpha detectors, the upgraded suite of instrumentation provides a wide range of analytical options to support research and mission needs.
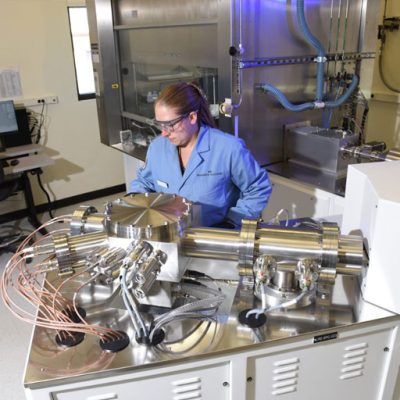
spectrometer installed in the Analytical Research Laboratory.
Construction of the Sample Preparation Lab: The Sample Preparation Laboratory (SPL) will focus on analyzing irradiated structural materials. It closes an identified nuclear energy research capability gap by greatly increasing sample throughput and nanoscale research capabilities. SPL will provide a central hub for the Department of Energy Office of Nuclear Energy research collaborations with its world-class instrumentation and ability to prepare, analyze and ship alpha-free materials to universities, industry partners and other DOE user facilities for research. The facility will be a nonreactor Hazard Category 3 nuclear facility designed to meet stringent seismic, vibration, electrical and magnetic field, acoustic, and temperature fluctuation requirements for advanced analytical equipment. It will support development and deployment of equipment instruments, and models to meet future nuclear material and fuel development RD&D needs over a 40-year period. The SPL will complete the suite of facilities fulfilling near-term advanced post-irradiation examination needs serving as a center for advanced fuels and materials characterization, as well as development of new processes, tools and instruments to further advanced nuclear energy research. Construction of the 49,000-square-foot, three-story SPL began in the spring of 2020, and all exterior building systems have been completed. Additional activities include interior construction, elevators, and work on the hot cells and shielded enclosures. When completed and operational in November 2026, the $166 million project will provide physical infrastructure and laboratory capabilities needed to satisfy world-class advanced post-irradiation examination capabilities to significantly advance nuclear energy RD&D objectives.
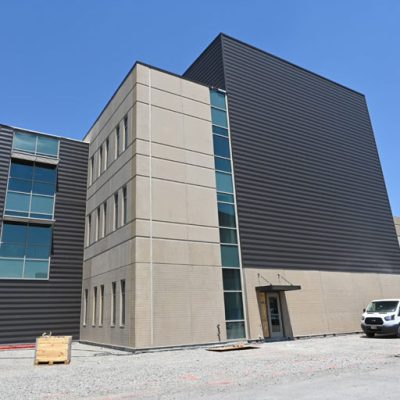
and Fuels Complex, will expand INL’s research capabilities
on nuclear structural materials.
Our core capabilities:
The Department of Energy (DOE) recognizes that our exemplary expertise with dedicated staff members, facilities and equipment span 16 of the 25 DOE core capabilities, and that we are developing two additional capabilities. These 18 core capabilities support our five S&T initiatives. Click through the icons below for official definitions of our core capabilities.
ADVANCED COMPUTER SCIENCE, VISUALIZATION, AND DATA
The ability to have a widely recognized role in advances in all applications in computational science and engineering. A core capability in these areas would involve expertise in areas such as programming languages, high-performance computing tools, peta- to exa-scale scientific data management and scientific visualization, distributed computing infrastructure, programming models for novel computer architectures, and automatic tuning for improving code performance, with unique and/or world-leading components in one or more of these areas. The capability requires access to (note: these resources do not need to be co-located) a high-end computational facility with the resources to test and develop new tools, libraries, languages, etc. In addition, linkages to application teams in computational science and/or engineering of interest to the Department of Energy and/or other Federal agencies would be beneficial to promptly address needs and requirements of those teams.
APPLIED MATERIALS SCIENCE AND ENGINEERING
The ability to conduct theoretical, experimental, and computational research to understand and characterize materials with focus on the design, synthesis, scale-up, prediction and measurement of structure/property relationships, the role of defects in controlling properties, the performance of materials in hostile environments to include mechanical behavior and long-term environmental stability, and the large-scale production of new materials with specific properties. The strong linkages with molecular science, engineering, and environmental science provides a basis for the development of materials that improve the efficiency, economy, cost-effectiveness, environmental acceptability, and safety in energy generation, conversion, transmission, and end-use technologies and systems. Primary supporting disciplines and field include materials synthesis, characterization, and processing; chemical and electrochemistry, combinatorial chemistry, surface science, catalysis, analytical and molecular science; and computation science.
BIOLOGICAL AND BIOPROCESS ENGINEERING
Applies understanding of complex biological systems and phenomena to design, prototype, test and validate processes components, technologies, and systems relevant to (1) bioenergy production, (2) environmental contaminants processing, and (3) global carbon cycling and biosequestration. Primary supporting disciplines include chemical engineering, agricultural science, fermentation science, materials science and engineering, and systems science.
CHEMICAL AND MOLECULAR SCIENCE (EMERGING)
The ability to conduct experimental, theoretical, and computational research to fundamentally understand chemical change and energy flow in molecular systems that provide a basis for the development of new processes for the generation, storage, and use of energy and for mitigation of the environmental impacts of energy use. Areas of research include atomic, molecular, and optical sciences; gas-phase chemical physics; condensed phase and interfacial molecular science; solar photochemistry; photosynthetic systems; physical biosciences; catalysis science; separation science; actinide chemistry; and geosciences.
CHEMICAL ENGINEERING
The ability to conduct applied chemical research that spans multiple scales from the molecular to macroscopic and from picoseconds to years. Chemical engineering translates scientific discovery into transformational solutions for advanced energy systems and other U.S. needs related to environment, security, and national competitiveness. The strong linkages between molecular, biological, and materials sciences, engineering science, and separations, catalysis and other chemical conversions provide a basis for the development of chemical processes that improve the efficiency, economy, competitiveness, environmental acceptability, and safety in energy generation, conversion, and utilization. A core capability in chemical engineering would underpin R&D in various areas such as nanomanufacturing, process intensification, biomass utilization, radiochemical processing, dielectric materials, advanced conducting materials, high-efficiency clean combustion, and would generate innovative solutions in alternative energy systems, carbon management, energy-intensive industrial processing, nuclear fuel cycle development, and waste and environmental management.
CONDENSED MATTER PHYSICS AND MATERIALS SCIENCE (EMERGING)
The ability to conduct experimental, theoretical, and computational research to fundamentally understand condensed matter physics and materials sciences that provide a basis for the development of materials that improve the efficiency, economy, environmental acceptability, and safety in energy generation, conversion, transmission, and utilization. Areas of research include experimental and theoretical condensed matter physics, x-ray and neutron scattering, electron and scanning probe microscopies, ultrafast materials science, physical and mechanical behavior of materials, radiation effects in materials, materials chemistry, and bimolecular materials.
CYBER AND INFORMATION SCIENCES
The disciplines, technologies, and practices designed to protect, analyze, and disseminate information from electronic sources, including computer systems, computer networks, and sensor networks. A core competency in this area would involve recognized expertise in one or more of the following topics: cyber security, information assurance, information analytics, knowledge representation, and information theory, control systems design and engineering, embedded systems, reverse engineering, and advanced hacking techniques. This core competency would be applied to: the protection of information systems and data from theft or attack; the collection, classification, analysis, and sharing of disparate data; and the creation of knowledge from heterogeneous information sources; securing control systems integrated into critical infrastructure; and increasing security, reliability, and resilience of automated processes and systems.
DECISION SCIENCE AND ANALYSIS
Derives knowledge and insights from measured and modeled data sets to further the understanding of and tradeoffs among resource and technology options, to identify and quantify the risks and impacts of current and emerging technologies on environmental systems, and to assess the impact of market dynamics, human behavior and regulations, policies or institutional practices on the development and uptake of technology. Primary supporting disciplines include engineering, environmental science, applied math, finance, business, social and political science, and market and behavioral economics. This capability provides credible and objective information to support DOE and others to support strategic planning and program direction, policy formulation and implementation, efforts to remove market barriers to deployment and engagement with stakeholders.
EARTH, ENVIRONMENTAL, AND ATMOSPHERIC SCIENCE
The ability to understand and predict the physical, chemical, and biological structure and function of subsurface environments to enable systems-level environmental prediction and decision support related to the sustainable development of subsurface resources, environmentally-responsible use of the subsurface for storage, and effective, mitigation of the impacts of environmental contamination from past nuclear weapons production and provide a scientific basis for the long-term stewardship of nuclear waste disposal via the integration of laboratory-specific research facilities, instrumentation and/or leadership-class computational systems, and multidisciplinary teams of individuals with expertise in environmental subsurface science and related disciplines in microbial ecology and biogeochemistry. This unique combination of tools and expertise is the foundation for research on (1) linking research across scales from the molecular to field scale, (2) integration of advanced computer models into the research and (3) multidisciplinary, iterative experimentation to understand and nutrient cycling and contaminant transport in complex subsurface environments. This ability can contribute to mitigating the impacts of environmental contamination from past nuclear weapons production and provide a scientific basis for the long-term stewardship of nuclear waste disposal, as well as understanding subsurface environments and their role in the functioning of terrestrial ecosystems.
LARGE-SCALE USER FACILITIES/ R&D FACILITIES/ADVANCED INSTRUMENTATION
The ability to conceive, design, construct and operate leading-edge specialty research facilities available to universities, industry, and national laboratories customers to conduct groundbreaking research and development activities and/or ‘at scale’ testing and demonstration of technology. This includes the ability to effectively manage construction of $100 million or greater one-of-a-kind scientific facilities, and to host hundreds to thousands of U.S. and international users in addition to carrying out world-class research at the facility itself. The ability to conceive, design, build, operate and use first-in-class technical instruments intended for a particular research purpose, often requiring the material expertise of multiple scientific disciplines. Instrumentation that can be created by a small number of individuals or that would sit on a laboratory benchtop is not considered part of this core capability.
MECHANICAL DESIGN AND ENGINEERING
Applies the principles of physics, mechanics, and materials science to analyze, design, test, validate, and enable operation of advanced engineered systems, machines, and tools. Includes equipment used to move or extract energy bearing materials (e.g., oil, gas, coal) or from moving fluids (e.g., water, wind, steam), as well as equipment used to convert energy to useful services (e.g., mobility, home heating and cooling, robotics, imaging devices, etc.) or to manufacture products. Primary supporting disciplines include physics, materials science, aerospace engineering, mechanical engineering, chemical engineering, electrical engineering, and computational science.
NUCLEAR AND RADIOCHEMISTRY
The ability to use a broad range of facilities, instrumentation, equipment and, often, interdisciplinary teams that apply the knowledge, data, methods, and techniques of nuclear chemistry, mechanical engineering, chemical engineering to missions of the Departments of Energy and Homeland Security. The elements of this capability are often brought together in unique combinations with those of other disciplines to address high priority needs such as new and improved nuclear systems; radioisotope production and advanced instrumentation for nuclear medicine; development of methods and systems to assure nonproliferation and combat terrorism; and environmental studies, monitoring, and remediation.
NUCLEAR ENGINEERING
The ability to use a broad range of facilities, instrumentation, equipment and, often, interdisciplinary teams that apply the knowledge, data, methods, and techniques of nuclear engineering, mechanical engineering, nuclear reactor physics, measurable science, and risk assessment to missions of the Departments of Energy and Homeland Security. The elements of this capability are often brought together in unique combinations with those of other disciplines to address high priority needs such as new and improved energy sources and systems; advanced instrumentation for nuclear systems; accelerator science and technology; and development of methods and systems to assure nonproliferation and combat terrorism.
POWER SYSTEMS AND ELECTRICAL ENGINEERING
Applies understanding of electromagnetic phenomena to design and engineer circuitry, electrical and electronic devices and equipment, sensors, instruments, and control systems to address the efficiency and reliability of power transmission and distribution systems, and the interface of the grid with variable generation and modern loads. Primary supporting disciplines include electrical engineering, power systems engineering, computational science, and materials synthesis, characterization, and processing.
SYSTEMS ENGINEERING AND INTEGRATION
The ability to solve problems holistically from the concept and design phase to ultimate deliverable and completion phase, by synthesizing multiple disciplines, and to develop and implement optimal solutions. The ability to develop solutions that address issues of national energy and environmental security. Areas of application of this capability include development of programs in energy supply, storage, transportation, and efficiency; and deployment of novel solutions to materials and sensor problems in fields of interest to the Department of Energy and/or the Department of Homeland Security.
COMPUTATIONAL SCIENCE
The ability to connect applied mathematics and computer science with research in scientific disciplines (e.g., biological sciences, chemistry, materials, physics, etc.). A core capability in this area involves expertise in applied mathematics, computer science and in scientific domains with a proven record of effectively and efficiently utilizing high performance computing resources to obtain significant results in areas of science and/or engineering of interest to the Department of Energy and/or other Federal agencies. The individual strengths in applied mathematics, computer science and in scientific domains in concert with the strength of the synergy between them is the critical element of this core capability.
ISOTOPE SCIENCE AND ENGINEERING
The ability to produce, process, enrich or separate isotopes for distribution, and the research that enables this core competency. This includes technologies, byproducts, and surplus materials for production and processing of radioisotopes using reactor or accelerator facilities, material separation, or enrichment of radioactive and stable isotopes. The ability to conduct theoretical, experimental, and computational research to develop isotope production, processing, enrichment, and separations capabilities and technologies, and to translate to routine production of new isotopes with specific assays. This core competency may include the production of isotopes following current Good Manufacturing Processes, and knowledge to operate reactors, particle accelerators, processing equipment or enrichment devices. Areas of research needed to develop this core competency include chemical processing, analytical chemistry, nuclear and radiochemical processing, catalysis science, separation science, material science, biology, actinide chemistry, computational science, reactor physics, accelerator science, advanced manufacturing, targetry, mechanical engineering, chemical engineering, robotics, enrichment science and engineering.
PLASMA AND FUSION ENERGY SCIENCES
The ability to control, maintain, and utilize matter in the state of plasma across a range of conditions to conduct world-leading research. This includes low-temperature plasmas which are utilized in applications such as microelectronics fabrication and nanomaterial synthesis and can have significant spinoff applications, and high temperature/high pressure plasmas, also known as burning plasmas, which are critical for developing the scientific foundation for fusion as a future energy source. This ability can be demonstrated across this entire range of plasma conditions in the operation of state-of-the-art experimental facilities that can support research on the fundamental physics of plasmas; in theory and advanced simulations which are critical to the full understanding of the plasma phenomena being studied and on how plasmas can be best controlled and utilized; and in enabling technologies that allow experiments to reach the necessary conditions where new discoveries can be made.