Science & Technology Overview
Advanced Materials and Manufacturing for Extreme Environments
Building on existing expertise in materials research and development for extreme environments with innovation in advanced manufacturing, INL is shifting the paradigm from design-build-test to digital design and manufacturing for nuclear fuels, lightweight materials and advanced survivability materials. Advanced manufacturing enables simultaneous fabrication with process monitoring and control, and supports all INL mission area needs.
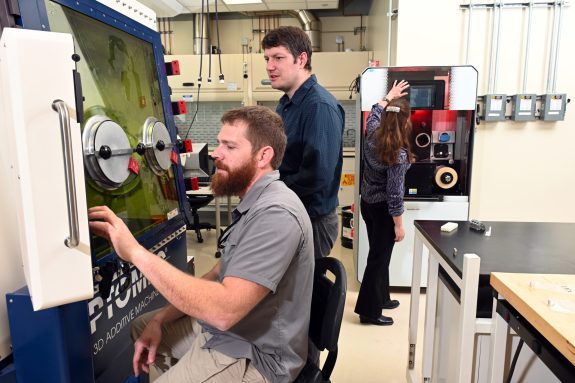
avenues of research into component manufacturing and materials discovery
INL develops and designs high-entropy alloys, high-temperature materials, metamaterials and radiation-resistant alloys as lightweight materials, next-generation reactor components and advanced survivability materials. INL is positioned to create advanced materials for extreme environments and strategically advance capabilities to relate process parameters to material performance.
A fundamental understanding of multiscale materials synthesis is needed to leverage advanced manufacturing techniques, including additive manufacturing, for material discovery and rapid fabrication of materials with highly tuned and tightly controlled properties. Coupling fundamental understanding with systems design techniques for rapid materials synthesis and processing enables fabrication and qualification of customized, high-performance materials in a single iteration. INL focuses on transformative materials design and advanced manufacturing for material functionality and durability in extreme environments. Looking ahead, INL is focused on a key component of material design capability to predict phases and phase transformations, leading to development of materials with specific microstructures and performance behavior. Traditionally, developing phase diagrams and measuring phase transformations and other kinetics information required extensive experimental campaigns. This method allows for developing phase diagrams and transforming new systems computationally, greatly reducing the cost and accelerating the speed of gathering this foundational information.
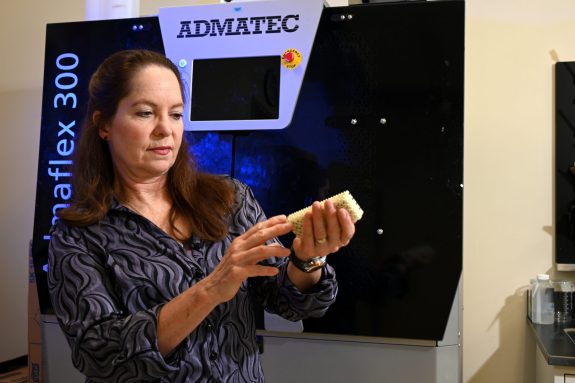
to additively manufacture ceramics or metals.
INL creates high-throughput experimental infrastructure combined with artificial intelligence to select and evaluate the broad array of material compositions that can be created using digital design and manufacturing. Rapid testing and characterization with deep process understanding drives the identification of important material properties and supports the verification and validation requirements for advanced materials in extreme environments.
One Laboratory Directed Research and Development (LDRD) project focuses on utilizing new high-throughput synthesis techniques to create arrays of different high-entropy alloys in parallel on standardized substrates. These arrays are then subjected to accelerator-based ion irradiation and subsequently characterized to experimentally screen for alloy compositions that show promising resistance to irradiation damage. Such parallelized synthesis capabilities, made possible through advanced manufacturing, will accelerate the discovery and development of novel materials for advanced nuclear applications more rapidly than with traditional metallurgy alone. INL seeks to realize the potential of these high-throughput techniques, which require strong integration of advanced manufacturing process development efforts, by integrating comprehensive data analytics and modeling.
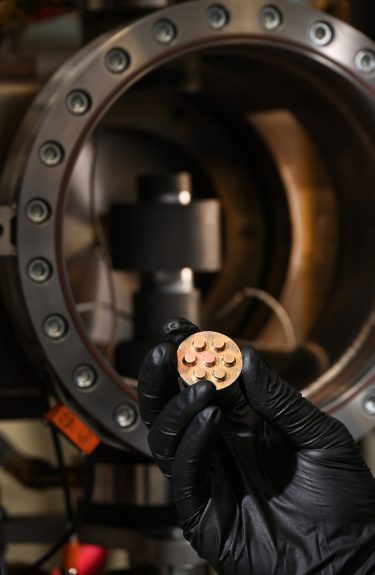
and characterization to screen for new alloys. Samples
were made using custom electric field assisted sintering
tooling and filler material to simultaneously
produce composite samples on a single substrate.
INL focuses on manufacturing materials with simultaneous on-the-fly process monitoring and adjustment. In situ process measurements verify and validate predictive models of advanced manufacturing processes and their associated material performance. In 2023, research will focus on the laser engineered net shaping and electric field assisted sintering manufacturing processes, material jetting/direct write, and digital light processing. Each technique spans varying length, time scales, geometries, print resolutions and material types.
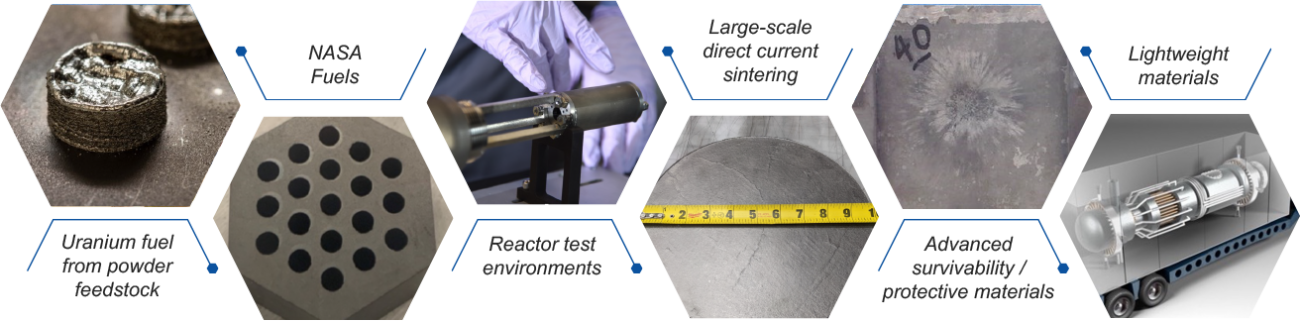
Predicting material performance requires experimenting, modeling, artificial intelligence and data analytics to relate the advanced manufacturing process parameters to the material properties. INL integrates multiphysics models with the full range of manufacturing systems, closing the loop between physical systems and multiphysics models to provide a foundation for next-generation, process-aware digital twin approaches. INL is leveraging existing capabilities and building a comprehensive set of tools to design materials for advanced manufacturing methods that will have long service lifetimes. INL teams are using machine learning to improve the descriptions of atomic interactions, meaning more accurate predictions of materials properties, from phase diagrams to radiation performance. They are also incorporating machine learning capabilities into the MOOSE framework, expanding abilities to model complex systems and efficiently obtain statistical information. Future research activities will focus on integrating multiscale mechanistic and machine learning models that support prediction of the performance of advanced manufacturing materials.
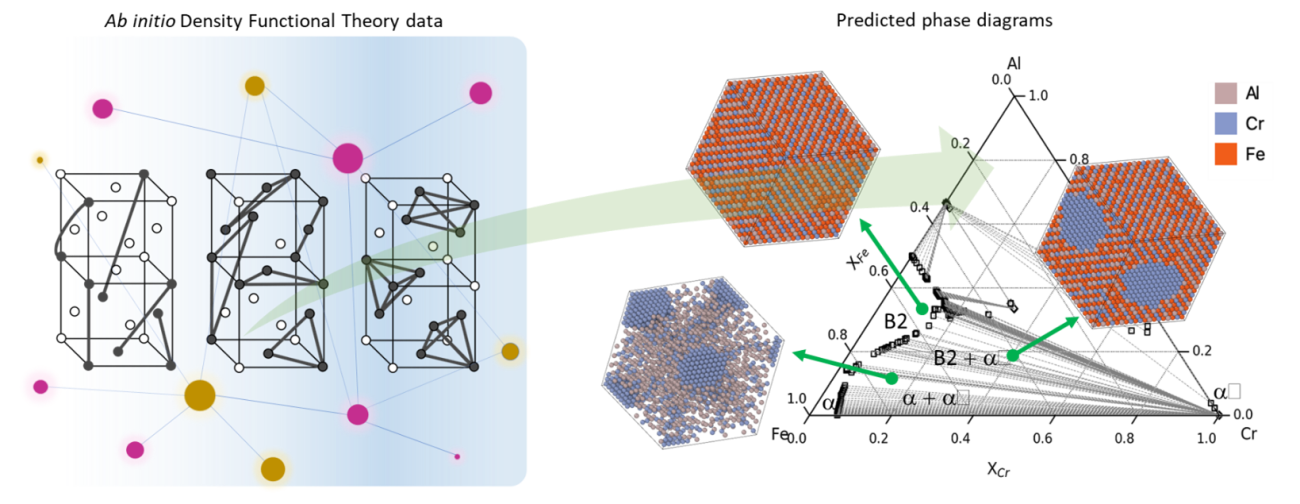
composition variation enables calculation of multicomponent phase diagrams without experimental data.
ADVANCED COMPUTER SCIENCE, VISUALIZATION, AND DATA
The ability to have a widely recognized role in advances in all applications in computational science and engineering. A core capability in these areas would involve expertise in areas such as programming languages, high-performance computing tools, peta- to exa-scale scientific data management and scientific visualization, distributed computing infrastructure, programming models for novel computer architectures, and automatic tuning for improving code performance, with unique and/or world-leading components in one or more of these areas. The capability requires access to (note: these resources do not need to be co-located) a high-end computational facility with the resources to test and develop new tools, libraries, languages, etc. In addition, linkages to application teams in computational science and/or engineering of interest to the Department of Energy and/or other Federal agencies would be beneficial to promptly address needs and requirements of those teams.
APPLIED MATERIALS SCIENCE AND ENGINEERING
The ability to conduct theoretical, experimental, and computational research to understand and characterize materials with focus on the design, synthesis, scale-up, prediction and measurement of structure/property relationships, the role of defects in controlling properties, the performance of materials in hostile environments to include mechanical behavior and long-term environmental stability, and the large-scale production of new materials with specific properties. The strong linkages with molecular science, engineering, and environmental science provides a basis for the development of materials that improve the efficiency, economy, cost-effectiveness, environmental acceptability, and safety in energy generation, conversion, transmission, and end-use technologies and systems. Primary supporting disciplines and field include materials synthesis, characterization, and processing; chemical and electrochemistry, combinatorial chemistry, surface science, catalysis, analytical and molecular science; and computation science.
BIOLOGICAL AND BIOPROCESS ENGINEERING
Applies understanding of complex biological systems and phenomena to design, prototype, test and validate processes components, technologies, and systems relevant to (1) bioenergy production, (2) environmental contaminants processing, and (3) global carbon cycling and biosequestration. Primary supporting disciplines include chemical engineering, agricultural science, fermentation science, materials science and engineering, and systems science.
CHEMICAL AND MOLECULAR SCIENCE (EMERGING)
The ability to conduct experimental, theoretical, and computational research to fundamentally understand chemical change and energy flow in molecular systems that provide a basis for the development of new processes for the generation, storage, and use of energy and for mitigation of the environmental impacts of energy use. Areas of research include atomic, molecular, and optical sciences; gas-phase chemical physics; condensed phase and interfacial molecular science; solar photochemistry; photosynthetic systems; physical biosciences; catalysis science; separation science; actinide chemistry; and geosciences.
CHEMICAL ENGINEERING
The ability to conduct applied chemical research that spans multiple scales from the molecular to macroscopic and from picoseconds to years. Chemical engineering translates scientific discovery into transformational solutions for advanced energy systems and other U.S. needs related to environment, security, and national competitiveness. The strong linkages between molecular, biological, and materials sciences, engineering science, and separations, catalysis and other chemical conversions provide a basis for the development of chemical processes that improve the efficiency, economy, competitiveness, environmental acceptability, and safety in energy generation, conversion, and utilization. A core capability in chemical engineering would underpin R&D in various areas such as nanomanufacturing, process intensification, biomass utilization, radiochemical processing, dielectric materials, advanced conducting materials, high-efficiency clean combustion, and would generate innovative solutions in alternative energy systems, carbon management, energy-intensive industrial processing, nuclear fuel cycle development, and waste and environmental management.
CONDENSED MATTER PHYSICS AND MATERIALS SCIENCE (EMERGING)
The ability to conduct experimental, theoretical, and computational research to fundamentally understand condensed matter physics and materials sciences that provide a basis for the development of materials that improve the efficiency, economy, environmental acceptability, and safety in energy generation, conversion, transmission, and utilization. Areas of research include experimental and theoretical condensed matter physics, x-ray and neutron scattering, electron and scanning probe microscopies, ultrafast materials science, physical and mechanical behavior of materials, radiation effects in materials, materials chemistry, and bimolecular materials.
CYBER AND INFORMATION SCIENCES
The disciplines, technologies, and practices designed to protect, analyze, and disseminate information from electronic sources, including computer systems, computer networks, and sensor networks. A core competency in this area would involve recognized expertise in one or more of the following topics: cyber security, information assurance, information analytics, knowledge representation, and information theory, control systems design and engineering, embedded systems, reverse engineering, and advanced hacking techniques. This core competency would be applied to: the protection of information systems and data from theft or attack; the collection, classification, analysis, and sharing of disparate data; and the creation of knowledge from heterogeneous information sources; securing control systems integrated into critical infrastructure; and increasing security, reliability, and resilience of automated processes and systems.
EARTH, ENVIRONMENTAL, AND ATMOSPHERIC SCIENCE
The ability to understand and predict the physical, chemical, and biological structure and function of subsurface environments to enable systems-level environmental prediction and decision support related to the sustainable development of subsurface resources, environmentally-responsible use of the subsurface for storage, and effective, mitigation of the impacts of environmental contamination from past nuclear weapons production and provide a scientific basis for the long-term stewardship of nuclear waste disposal via the integration of laboratory-specific research facilities, instrumentation and/or leadership-class computational systems, and multidisciplinary teams of individuals with expertise in environmental subsurface science and related disciplines in microbial ecology and biogeochemistry. This unique combination of tools and expertise is the foundation for research on (1) linking research across scales from the molecular to field scale, (2) integration of advanced computer models into the research and (3) multidisciplinary, iterative experimentation to understand and nutrient cycling and contaminant transport in complex subsurface environments. This ability can contribute to mitigating the impacts of environmental contamination from past nuclear weapons production and provide a scientific basis for the long-term stewardship of nuclear waste disposal, as well as understanding subsurface environments and their role in the functioning of terrestrial ecosystems.
LARGE-SCALE USER FACILITIES/ R&D FACILITIES/ADVANCED INSTRUMENTATION
The ability to conceive, design, construct and operate leading-edge specialty research facilities available to universities, industry, and national laboratories customers to conduct groundbreaking research and development activities and/or ‘at scale’ testing and demonstration of technology. This includes the ability to effectively manage construction of $100 million or greater one-of-a-kind scientific facilities, and to host hundreds to thousands of U.S. and international users in addition to carrying out world-class research at the facility itself. The ability to conceive, design, build, operate and use first-in-class technical instruments intended for a particular research purpose, often requiring the material expertise of multiple scientific disciplines. Instrumentation that can be created by a small number of individuals or that would sit on a laboratory benchtop is not considered part of this core capability.
MECHANICAL DESIGN AND ENGINEERING
Applies the principles of physics, mechanics, and materials science to analyze, design, test, validate, and enable operation of advanced engineered systems, machines, and tools. Includes equipment used to move or extract energy bearing materials (e.g., oil, gas, coal) or from moving fluids (e.g., water, wind, steam), as well as equipment used to convert energy to useful services (e.g., mobility, home heating and cooling, robotics, imaging devices, etc.) or to manufacture products. Primary supporting disciplines include physics, materials science, aerospace engineering, mechanical engineering, chemical engineering, electrical engineering, and computational science.
NUCLEAR ENGINEERING
The ability to use a broad range of facilities, instrumentation, equipment and, often, interdisciplinary teams that apply the knowledge, data, methods, and techniques of nuclear engineering, mechanical engineering, nuclear reactor physics, measurable science, and risk assessment to missions of the Departments of Energy and Homeland Security. The elements of this capability are often brought together in unique combinations with those of other disciplines to address high priority needs such as new and improved energy sources and systems; advanced instrumentation for nuclear systems; accelerator science and technology; and development of methods and systems to assure nonproliferation and combat terrorism.
POWER SYSTEMS AND ELECTRICAL ENGINEERING
Applies understanding of electromagnetic phenomena to design and engineer circuitry, electrical and electronic devices and equipment, sensors, instruments, and control systems to address the efficiency and reliability of power transmission and distribution systems, and the interface of the grid with variable generation and modern loads. Primary supporting disciplines include electrical engineering, power systems engineering, computational science, and materials synthesis, characterization, and processing.
SYSTEMS ENGINEERING AND INTEGRATION
The ability to solve problems holistically from the concept and design phase to ultimate deliverable and completion phase, by synthesizing multiple disciplines, and to develop and implement optimal solutions. The ability to develop solutions that address issues of national energy and environmental security. Areas of application of this capability include development of programs in energy supply, storage, transportation, and efficiency; and deployment of novel solutions to materials and sensor problems in fields of interest to the Department of Energy and/or the Department of Homeland Security.
COMPUTATIONAL SCIENCE
The ability to connect applied mathematics and computer science with research in scientific disciplines (e.g., biological sciences, chemistry, materials, physics, etc.). A core capability in this area involves expertise in applied mathematics, computer science and in scientific domains with a proven record of effectively and efficiently utilizing high performance computing resources to obtain significant results in areas of science and/or engineering of interest to the Department of Energy and/or other Federal agencies. The individual strengths in applied mathematics, computer science and in scientific domains in concert with the strength of the synergy between them is the critical element of this core capability.