Science & Technology Overview
Integrated Fuel Cycle Solutions
INL nuclear fuel cycle capabilities enable the deployment of technologies that sustain the current reactor fleet, support demonstration and deployment of new advanced reactors, and facilitate management and disposition of existing and future radiological waste materials. INL focuses on deploying nuclear energy systems with confidence by decreasing proliferation risk through research that demonstrates process transparency and supports safeguards and security by design.
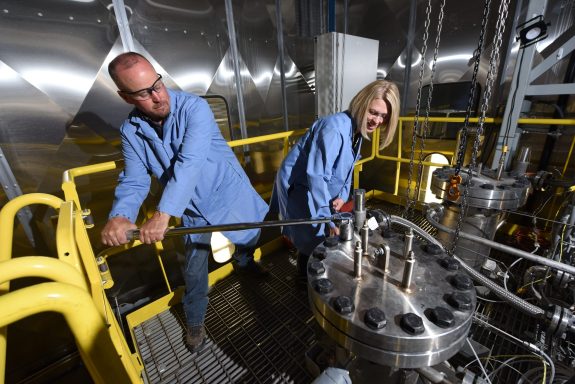
demonstration and development (RD&D) facility perform work that will inform
dry decladding of used nuclear fuel samples to support recovery
and downblending of highly enriched uranium to
high-assay low-enriched uranium.
INL ensures the domestic supply of special nuclear material and strategic isotopes for industrial and medical use. Many next-generation advanced reactors will use high-assay low-enriched uranium fuel, called HALEU, which contains 5 to 20% uranium-235. INL leads Department of Energy (DOE) RD&D efforts to support the U.S. near-term HALEU fuel cycle infrastructure by providing an interim supply from recovered and downblended highly enriched uranium from Experimental Breeder Reactor-II used nuclear fuel and other highly enriched uranium stocks. INL develops technologies to facilitate the HALEU supply and works with industry and DOE to ensure meeting private sector fuel market requirements that support future advanced reactor developers while honoring INL’s commitment to the state of Idaho to stabilize EBR-II used fuel by 2028. INL also develops industrial and medical isotopes. Under DOE’s Office of Science Isotope Research Development and Production Program, INL produces cobalt-60, with applications spanning medical radiation therapy, medical and food sterilization, and other industrial applications.
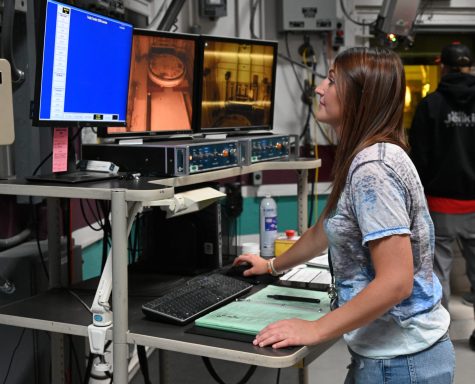
transfer as part of EBR-II fuel reprocessing and HALEU development.
INL integrates waste minimization and management into advanced reactor fuel cycle technologies by discovering methods to minimize waste while reducing operating and maintenance costs. Researchers are developing modeling tools to ensure safe and compliant extended dry storage for the existing light water reactor used nuclear fuel, in addition to new tools developed through Laboratory Directed Research and Development investments to support advanced reactor spent fuel disposition and predictive capabilities for future repository programs.
To address technical gaps related to mixed waste streams that contain radioactive and chemical hazards, INL is developing waste management test bed capabilities at the Materials and Fuels Complex. This will address challenges associated with legacy and newly generated waste streams. In partnership with industry, INL successfully treated an unirradiated EBR-II blanket material subassembly, showing that reactivity characteristics can be removed while generating a product acceptable for land disposal. This technology is being evaluated for treating waste streams from future reactor programs. In addition, based on INL’s conclusion that aluminum-clad used nuclear fuel can be safely and viably dry stored for more than 50 years, INL is expanding options to deploy road-ready dry storage of used nuclear fuel at the INL Site.
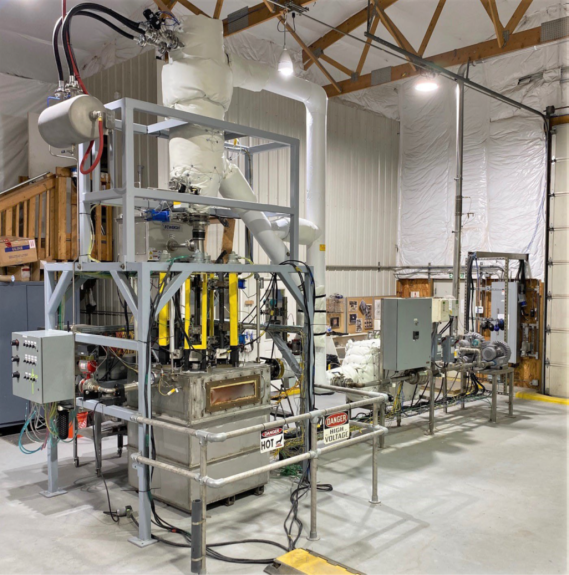
scale units and engineering scale unit have been deployed to
demonstrate and treat reactive mixed waste streams.
Innovating a fuel cycle with inherent process transparency reduces proliferation risk. INL incorporates safeguards by design to monitor nuclear materials throughout the fuel cycle. INL augments data analytics with artificial intelligence while also pursuing novel concepts in automated multisensor data analytics for fuel cycle safeguards and nonproliferation. Advanced reactor designs are being developed with systems that differ from traditional light water reactor material accountancy. INL is demonstrating innovative safeguards and security concepts, applicable to advanced reactors and their fuel cycles to meet national security objectives, and works with the International Atomic Energy Agency to incorporate international safeguards and security requirements.
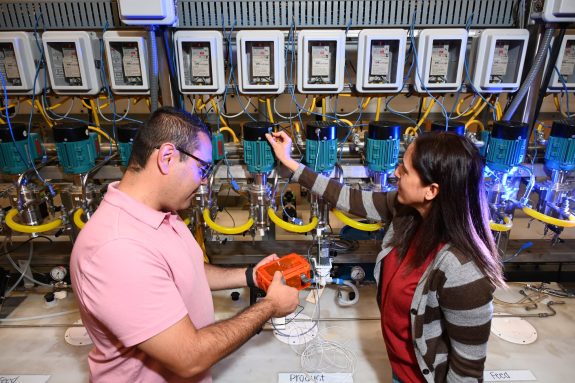
for monitoring solvent extraction equipment for their Laboratory Directed Research
and Development project, which could enhance safeguarding of special nuclear materials.
INL configures fuel cycle test bed facilities to provide a modern, flexible RD&D test environment. These facilities incorporate engineering and pilot-scale equipment, modeling, simulation, data science and cyber tools to address science and technology gaps. Advanced test beds help INL lead advanced reactor development, including small modular reactors, microreactors, liquid-fuel reactors and TRISO-fuel development, and to advance unique capabilities to address DOE special nuclear material legacy issues. All new fuel cycle test beds are designed to demonstrate innovative safeguards and security concepts applicable to advanced reactors and their fuel cycles.
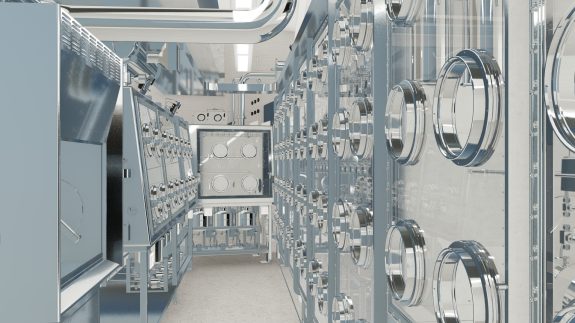
platform for demonstrating new safeguards and security concepts applicable
to advanced fuel cycle operations.
Stewarding RD&D test bed facilities is critical for INL to engage and train the next generation of fuel cycle, nonproliferation and waste management experts. Fuel cycle test beds serve as user facilities that allow multiple programs and mission partner agencies to run experiments that explore scientific and technology issues, and develop data needed to address technical questions associated with evolving fuel cycles.
APPLIED MATERIALS SCIENCE AND ENGINEERING
The ability to conduct theoretical, experimental, and computational research to understand and characterize materials with focus on the design, synthesis, scale-up, prediction and measurement of structure/property relationships, the role of defects in controlling properties, the performance of materials in hostile environments to include mechanical behavior and long-term environmental stability, and the large-scale production of new materials with specific properties. The strong linkages with molecular science, engineering, and environmental science provides a basis for the development of materials that improve the efficiency, economy, cost-effectiveness, environmental acceptability, and safety in energy generation, conversion, transmission, and end-use technologies and systems. Primary supporting disciplines and field include materials synthesis, characterization, and processing; chemical and electrochemistry, combinatorial chemistry, surface science, catalysis, analytical and molecular science; and computation science.
CHEMICAL ENGINEERING
The ability to conduct applied chemical research that spans multiple scales from the molecular to macroscopic and from picoseconds to years. Chemical engineering translates scientific discovery into transformational solutions for advanced energy systems and other U.S. needs related to environment, security, and national competitiveness. The strong linkages between molecular, biological, and materials sciences, engineering science, and separations, catalysis and other chemical conversions provide a basis for the development of chemical processes that improve the efficiency, economy, competitiveness, environmental acceptability, and safety in energy generation, conversion, and utilization. A core capability in chemical engineering would underpin R&D in various areas such as nanomanufacturing, process intensification, biomass utilization, radiochemical processing, dielectric materials, advanced conducting materials, high-efficiency clean combustion, and would generate innovative solutions in alternative energy systems, carbon management, energy-intensive industrial processing, nuclear fuel cycle development, and waste and environmental management.
EARTH, ENVIRONMENTAL, AND ATMOSPHERIC SCIENCE
The ability to understand and predict the physical, chemical, and biological structure and function of subsurface environments to enable systems-level environmental prediction and decision support related to the sustainable development of subsurface resources, environmentally-responsible use of the subsurface for storage, and effective, mitigation of the impacts of environmental contamination from past nuclear weapons production and provide a scientific basis for the long-term stewardship of nuclear waste disposal via the integration of laboratory-specific research facilities, instrumentation and/or leadership-class computational systems, and multidisciplinary teams of individuals with expertise in environmental subsurface science and related disciplines in microbial ecology and biogeochemistry. This unique combination of tools and expertise is the foundation for research on (1) linking research across scales from the molecular to field scale, (2) integration of advanced computer models into the research and (3) multidisciplinary, iterative experimentation to understand and nutrient cycling and contaminant transport in complex subsurface environments. This ability can contribute to mitigating the impacts of environmental contamination from past nuclear weapons production and provide a scientific basis for the long-term stewardship of nuclear waste disposal, as well as understanding subsurface environments and their role in the functioning of terrestrial ecosystems.
LARGE-SCALE USER FACILITIES/ R&D FACILITIES/ADVANCED INSTRUMENTATION
The ability to conceive, design, construct and operate leading-edge specialty research facilities available to universities, industry, and national laboratories customers to conduct groundbreaking research and development activities and/or ‘at scale’ testing and demonstration of technology. This includes the ability to effectively manage construction of $100 million or greater one-of-a-kind scientific facilities, and to host hundreds to thousands of U.S. and international users in addition to carrying out world-class research at the facility itself. The ability to conceive, design, build, operate and use first-in-class technical instruments intended for a particular research purpose, often requiring the material expertise of multiple scientific disciplines. Instrumentation that can be created by a small number of individuals or that would sit on a laboratory benchtop is not considered part of this core capability.
MECHANICAL DESIGN AND ENGINEERING
Applies the principles of physics, mechanics, and materials science to analyze, design, test, validate, and enable operation of advanced engineered systems, machines, and tools. Includes equipment used to move or extract energy bearing materials (e.g., oil, gas, coal) or from moving fluids (e.g., water, wind, steam), as well as equipment used to convert energy to useful services (e.g., mobility, home heating and cooling, robotics, imaging devices, etc.) or to manufacture products. Primary supporting disciplines include physics, materials science, aerospace engineering, mechanical engineering, chemical engineering, electrical engineering, and computational science.
NUCLEAR AND RADIOCHEMISTRY
The ability to use a broad range of facilities, instrumentation, equipment and, often, interdisciplinary teams that apply the knowledge, data, methods, and techniques of nuclear chemistry, mechanical engineering, chemical engineering to missions of the Departments of Energy and Homeland Security. The elements of this capability are often brought together in unique combinations with those of other disciplines to address high priority needs such as new and improved nuclear systems; radioisotope production and advanced instrumentation for nuclear medicine; development of methods and systems to assure nonproliferation and combat terrorism; and environmental studies, monitoring, and remediation.
NUCLEAR ENGINEERING
The ability to use a broad range of facilities, instrumentation, equipment and, often, interdisciplinary teams that apply the knowledge, data, methods, and techniques of nuclear engineering, mechanical engineering, nuclear reactor physics, measurable science, and risk assessment to missions of the Departments of Energy and Homeland Security. The elements of this capability are often brought together in unique combinations with those of other disciplines to address high priority needs such as new and improved energy sources and systems; advanced instrumentation for nuclear systems; accelerator science and technology; and development of methods and systems to assure nonproliferation and combat terrorism.
SYSTEMS ENGINEERING AND INTEGRATION
The ability to solve problems holistically from the concept and design phase to ultimate deliverable and completion phase, by synthesizing multiple disciplines, and to develop and implement optimal solutions. The ability to develop solutions that address issues of national energy and environmental security. Areas of application of this capability include development of programs in energy supply, storage, transportation, and efficiency; and deployment of novel solutions to materials and sensor problems in fields of interest to the Department of Energy and/or the Department of Homeland Security.
ISOTOPE SCIENCE AND ENGINEERING
The ability to produce, process, enrich or separate isotopes for distribution, and the research that enables this core competency. This includes technologies, byproducts, and surplus materials for production and processing of radioisotopes using reactor or accelerator facilities, material separation, or enrichment of radioactive and stable isotopes. The ability to conduct theoretical, experimental, and computational research to develop isotope production, processing, enrichment, and separations capabilities and technologies, and to translate to routine production of new isotopes with specific assays. This core competency may include the production of isotopes following current Good Manufacturing Processes, and knowledge to operate reactors, particle accelerators, processing equipment or enrichment devices. Areas of research needed to develop this core competency include chemical processing, analytical chemistry, nuclear and radiochemical processing, catalysis science, separation science, material science, biology, actinide chemistry, computational science, reactor physics, accelerator science, advanced manufacturing, targetry, mechanical engineering, chemical engineering, robotics, enrichment science and engineering.