Seventy-five years ago, the sweeping landscape of the Idaho desert over the Snake River Aquifer was a land of sagebrush, brown grass and lava rock. Today, the desert site facilities of Idaho National Laboratory are still home to sagebrush, brown grass and lava rock but also to of some of the world’s most cutting-edge nuclear fuel research and reactor prototype development.
Long-term efforts to advance nuclear technologies, which are a key part of the nation’s resilient and secure energy future, have historically centered around two main approaches: prototype demonstrations and irradiation testing. The National Reactor Testing Station (NRTS) was established in the desert west of Idaho Falls in 1949 as the ideal location to pursue both approaches.
Prototypes and demonstration reactors, such as the historic Experimental Breeder Reactor-I, are an excellent way to develop a specific reactor type. At the Site, this mission virtually disappeared for decades, but it has returned to the forefront of current efforts to develop advanced reactors.
In contrast, the irradiation testing mission has endured, uninterrupted, since the earliest days of NRTS. This mission is vital in support of U.S. national security and energy security goals to provide reliable, base-load generation capacity.
In the early days of nuclear energy research, most research reactors, which serve as neutron sources for irradiation testing, operated at low power levels, usually in the kilowatt range or less. The same is still true today. But nuclear researchers understood as early as 1947 that the world needed a new type of high-performance research reactor to enable rapid testing of fuel and material samples under the intense gamma and neutron environment they would experience in a commercial or Navy propulsion reactor’s core. The data from this type of high-performance research reactor would enable the nuclear industry to develop and license new reactors and improve the performance of existing ones.
Thus, the concept of the “high flux” reactor was born, driven by the rapid pace of Atomic Energy Commission prototyping, naval nuclear propulsion, and commercial power reactor development in the 1950s and 1960s.
What is Neutron Flux?
Reactor environments include heat and millions upon billions of neutrons flying through and around the reactor core – called flux – which affect the fuel and structural materials of the core. Test reactors are designed to intensify and reflect this flux into experiment locations to gather vital data on fuel and material performance.
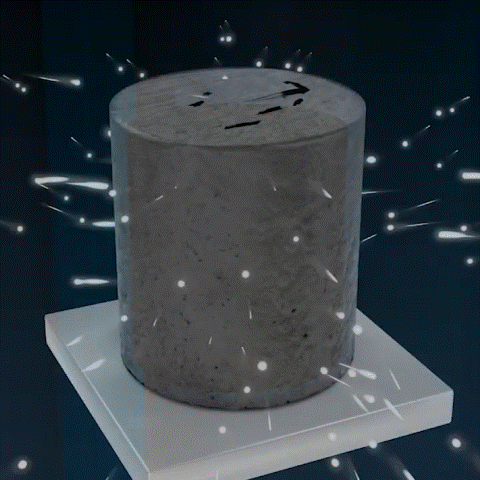
Materials Testing Reactor
The first high-flux test reactor had a working title during conceptual development that literally matched its mission – the Reactor Development Reactor. Clinton Laboratories (now Oak Ridge National Laboratory) scientists originally assumed the reactor would be built at their facilities in Tennessee. But officials soon decided that it made more sense to locate the world’s first test reactor – soon named Materials Testing Reactor (MTR) – at the new National Reactor Testing Station in Idaho alongside dozens of planned new prototype reactors. The demand for its capabilities drove a construction schedule that was incredibly fast by today’s standards. Groundbreaking in May 1950 to first criticality took only 22 months. Details of the reactor’s design were resolved on the fly as construction progressed. The project had a working budget of $40 million (over $500 million in today’s dollars), the largest such project ever launched in Idaho at the time.
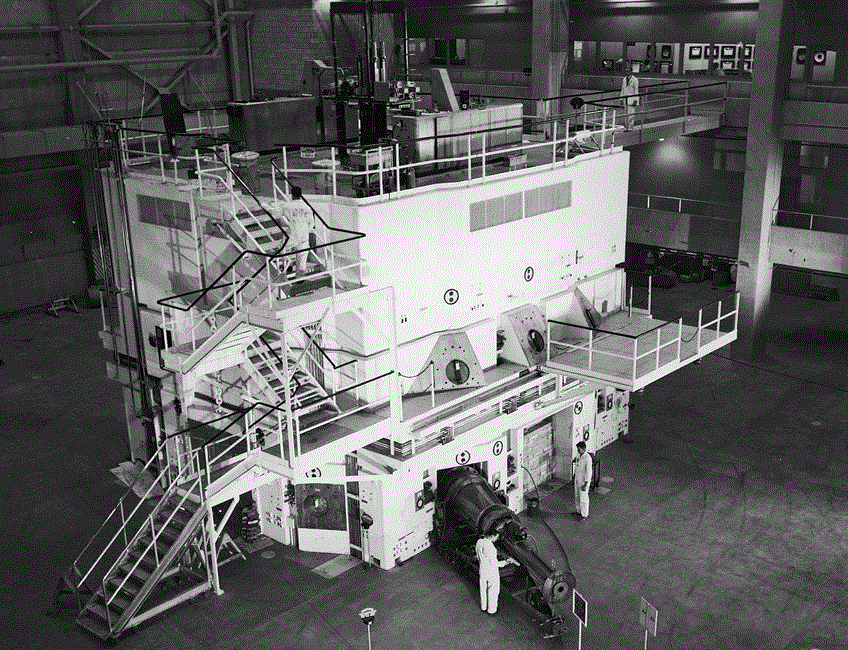
MTR boasted more than 100 testing positions — spaces in or around a reactor’s core into which material or fuel experiments can be placed — located around the reactor vessel and neutron beam tubes for additional research. With a design power of 30 megawatts, MTR could generate a neutron flux far higher than any research reactor then in existence. Even so, to keep pace with reactor development, MTR was uprated to 40 megawatts in 1955.
Engineering Test Reactor
The feverish pace of nuclear technology development quickly outgrew MTR’s capabilities. Proposed nuclear technologies required even higher flux levels and larger test spaces to obtain the necessary data. This need gave rise to the 175-megawatt Engineering Test Reactor (ETR), which was located just south of MTR in what was then known as the Test Reactor Area.
ETR achieved its first criticality on Sept. 19, 1957, and would continue operating until 1981. In addition to higher flux – up to four times greater than MTR – and testing positions that allowed for larger experiments, this second-generation test reactor featured numerous improvements. One of the most important improvements was the incorporation of experiment conditions inside the core in “inpile” tubes that could enable the highest possible flux levels in a separate controlled environment. This approach was more costly and slower but yielded much more reliable data, which enabled continued research and development for reactor designers.
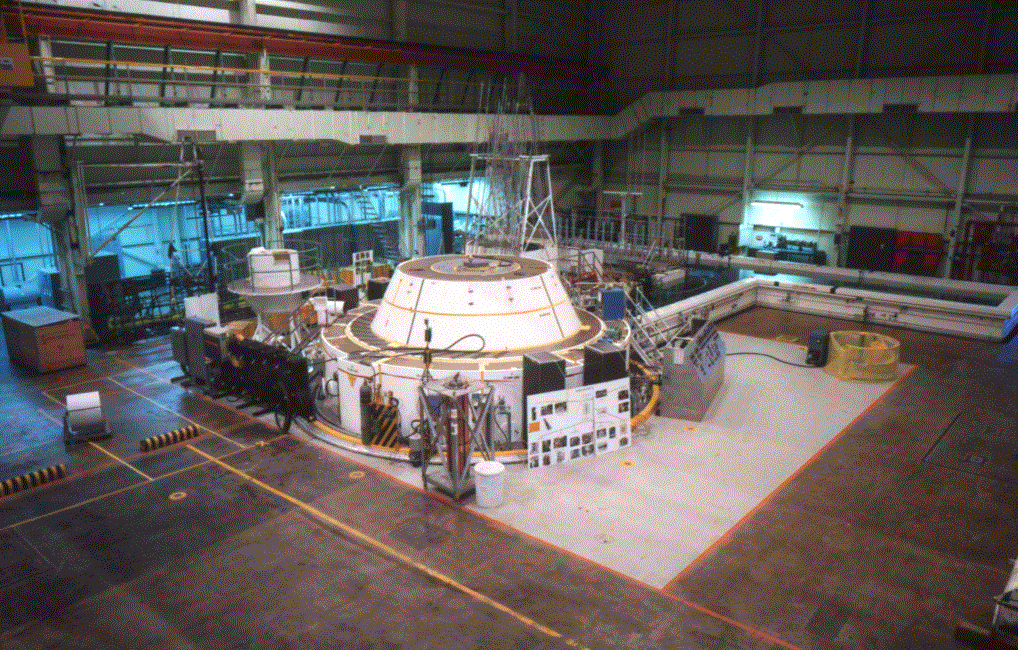
In the nuclear world, “pile” is another name for the reactor’s core – drawn from the first nuclear reactor, Chicago Pile-1. Inpile tubes are long pipes inside the reactor’s core that provide the most valuable test positions. Experimental fuels and materials can be exposed to intense neutron and gamma radiation to gather data on its performance.
But just a few years later, nuclear innovators outgrew ETR. In 1959, conceptual design work began on a third-generation high-performance research reactor to support the growing demand.
Advanced Test Reactor
Both Naval and commercially developed core designs continued to drive the need for more test positions with even higher flux. Despite their complexity, experiment loops, which provide the ability to independently control test variables like coolant material, temperature, pressure and flow rates, were in great demand since they produced much more reliable data about fuel performance in real-world conditions when compared with open drop-in test positions.
Deslonde deBoisblanc, an NRTS scientist with no physics doctorate but an instinctive understanding of neutron behavior, came up with a brilliant concept that addressed all the reactor core design needs and raised peak power to 250 megawatts. His elegant cloverleaf core design weaved the fuel in the core around nine large test positions, each of which could accommodate an individually controlled environment for a specific reactor type. The design also allowed for 68 other test positions in and around the core. Rotating control cylinders instead of control rods allowed consistent and precise control of power levels in each “lobe,” or corner, of the reactor core, allowing the Advanced Test Reactor (ATR) to perform like several different reactors in one.
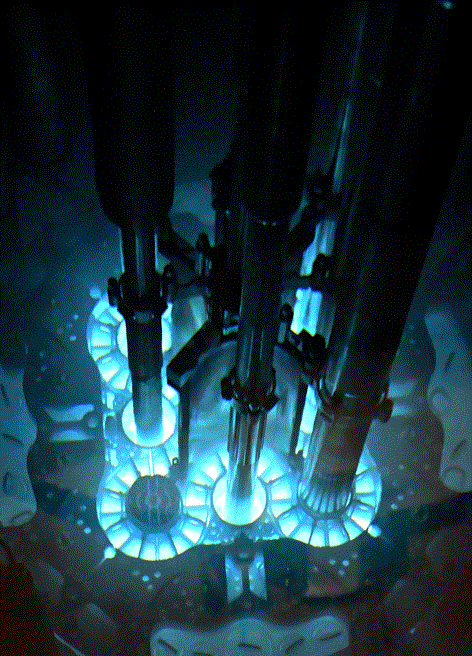
The design was far ahead of its time; ATR quickly became a foundational part of U.S. nuclear research infrastructure. Its capabilities provide integral support for the Navy’s national security mission and the development of current and advanced reactors. Even after 58 years, it remains the world’s most powerful, highest capacity and most versatile test reactor.
“As we focus on maintaining and improving ATR’s capabilities, it’s important to remember that we act as a team with the rest of INL. Irradiating experiments is important, but equally so are the fabrication and examination capabilities at INL and our research labs in Idaho Falls,” said Sean O’Kelly, associate lab director for the ATR Complex. “While MTR and ETR are long gone, ATR’s key customers have indicated a continued demand for its capabilities for at least another six decades. We’re working with them every day to help ensure we can continue our missions well into the future.“